
2D Materials
A Summary of 2D Materials
“2D materials” is a term used to describe a large, relatively new family of exciting two-dimensional molecular structures. Interest in these materials has exploded since the isolation of graphene from graphite in 2004, for their potential applications in science and engineering. Each 2D material represents a distinct chemical composition with unique properties and applications, and the chemistry of 2D materials spans across most of the periodic table. While 2D materials are diverse in their chemical makeup, they are unified by their characteristic shape, in which atoms bond together to form flat, individual molecular sheets no more than a few nanometers thick. Due to their unique shape and chemical properties, these molecular sheets have the potential to be light, strong, and adaptable to a wide number of applications in electronics, energy production, separations, chemistry, and sensor technology.
Graphene
Graphene is the most well-known and widely-publicized 2D material in existence today. The existence and properties of graphene as a distinct material have been theorized and even observed since 1947. However, it was not until 2004 that researchers at the University of Manchester were able to isolate the material for the first time by peeling single layers of graphene from a block of graphite using sticky tape. This deceptively simple method for producing graphene birthed the field of 2D materials research and won the 2010 Nobel Prize in Physics. Graphene has since been hailed as the next wonder material, and potentially disruptive technology in the fields of electronics, energy, health, construction and separations. As a testament to its significance, in 2013 the EU committed €1 bn to graphene research between 2013-23; over 10,000 academic papers have been published on graphene since 2005, and in 2012 BASF estimated the market for graphene to be worth $7.5 bn by 2025.
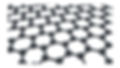
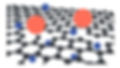
A visual representation of the atomic structure of graphene.
The permeability of pure graphene can be controlled through the addition of chemically modified nanopores, turning impermeable graphene into an effective material for separations.
Chemically, graphene is composed of carbon atoms bonded together in a hexagonal lattice, similar to an atomic-scale poultry netting. Each carbon in graphene represents a link in the fence and is bonded to four other carbons to form a flat, two-dimensional sheet of carbon atoms just one atom thick, as seen in Figure above. Because of this unique chemical structure, a single sheet of pure graphene conducts electricity better than copper, is both stronger and lighter than steel, is almost entirely transparent, and remains impermeable to all liquids and gases. While these properties are impressive in themselves, graphene also allows for control of its properties through physical and chemical alterations to its structure, making it a versatile platform for further development. For example, the permeability of graphene can be controlled by drilling nanopores into the graphene surface, allowing for separations by size exclusion and electrostatic interactions depending on the pore size, chemical composition of the pore edge, as seen in Figure above.
Applications of Graphene
For these reasons, graphene is expected to lead to significant advances in a diverse range of applications in the coming years. Some of the most notable anticipated advances are highlighted below:

In the fields of construction and manufacturing, graphene’s is expected to significantly improve the weight and durability of composite materials such as resins, rubber, concrete and protective coatings.

In the fields of electronics and energy storage, graphene’s low cost and high electrical conductivity are expected to allow for the production of flexible electronics, printable ink circuits, improved OLED displays, supercapacitors, improved chemical sensors and consumer batteries with improved storage capacities and charge times.
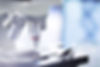
In the biomedical field, graphene is expected to allow for the development of DIY health testing kits, improve brain penetration, targeted drug delivery and smart implants due to its large surface area, electrical properties and flexibility in chemical modification.
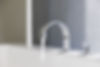
In the fields of separations and desalination, graphene represents the potential for significantly improved separation membrane technologies. Models predict that nanoporous graphene membranes could allow for water processing several hundred times faster than modern reverse osmosis membranes, with significant energy savings.
There are still hurdles that must be overcome to transition from conventional technologies to graphene-based alternatives, though an increasing number of graphene-based products are already entering market. Notable examples at this time include graphene composite sporting goods (e.g. tennis rackets and bicycle frames), graphene-based touch screen displays, gas sensors and sample grids for high performance microscopes. As graphene manufacturing becomes more sophisticated, more and more graphene-based applications are expected to become commercially viable, making graphene a must-watch material for the future.